Topological superconductivity has opened a new field of quantum matter where exotic particles arise from electron group behavior. Through their special safety mechanisms, my investigations of topological edge states have shown how these systems can transform quantum computing. Majorana zero modes observed at topological superconductors’ margins constitute a quantum physics revolution. Unprecedentedly precise techniques for controlling and manipulating these exotic quantum states have been shown recently in tests New materials with strong topological superconductivity at ever-more-practical temperatures have been created by scientists The field generates protected quantum states by combining useful materials science with strong mathematical ideas. These devices present a viable stage for the development of fault-tolerant quantum computers. Topological superconductors have helped to expose surprising links between condensed matter and particle physics. Quantum computers with inherent error protection could be made possible by the technologies.
Table of Contents
Quantum Dance at the Edge
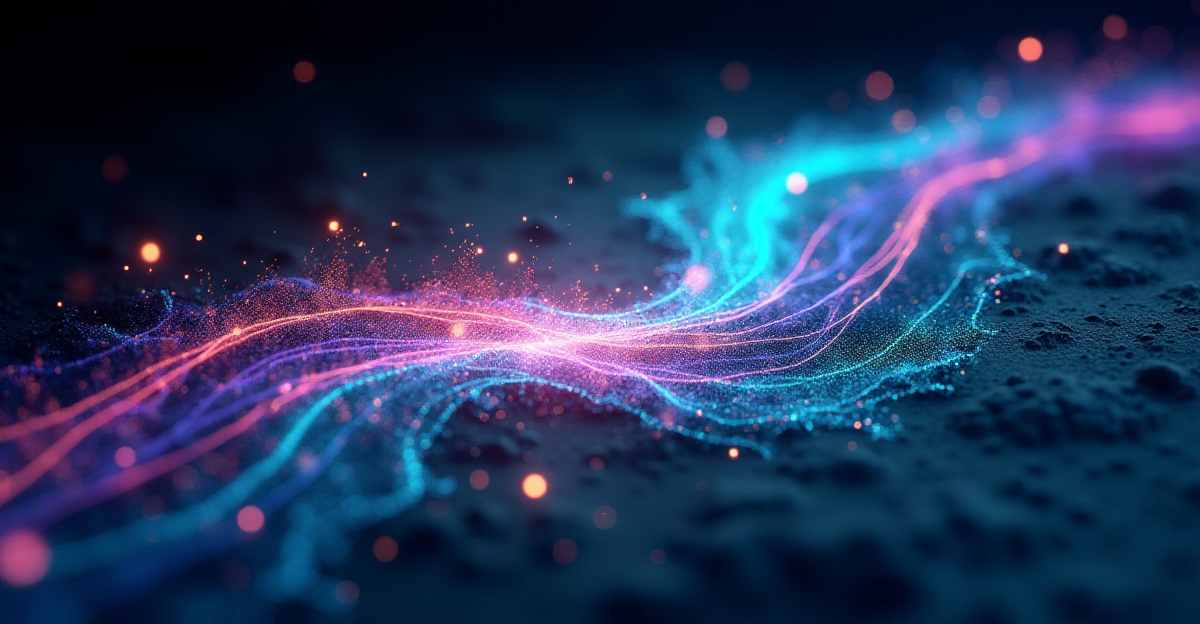
For some considerable time, the discipline of topological superconductivity has captivated me. Research in this fascinating field links the apparently separate domains of abstract mathematics and physical materials science. The fundamental idea centers on the odd behavior of electrons inside some superconducting materials that results in the formation of exotic quantum states sometimes described as Majorana modes. These are not your normal electrons; they have special qualities that might transform the discipline of quantum computing. Imagine a world in which the mistakes afflicting present technology are absent from quantum computers. With “topological superconductivity,” this is the promise and a very fascinating potential. My work is on the edge states inside these materials, the limits where these Majorana modes live and dance, so challenging our conventional wisdom of physics. These edge states are remarkably strong and provide a degree of security not possible years before. Perched on the brink of a material, they are like little, strong dancers executing their complex motions free from the noise and interference that might readily disturb more traditional quantum systems. This resilience has great consequences and presents the possibility of creating quite dependable and robust quantum computers. The construction of fault-tolerant quantum computers, able of processing data with until unheard-of accuracy, depends on this stability. The interaction of the basic ideas with useful applications fascinates me absolutely. New material design and synthesis help to bring to life the apparently arcane character of topological superconductivity.
Many of the present studies are motivated by the hunt for useful applications of topological superconductivity. Approaching realization of its technological potential, scientists are creating novel materials exhibiting strong topological superconductivity at even higher temperatures. This is a crucial step since present systems are problematic for general usage since they sometimes demand quite low temperatures. Crucially also is our capacity to govern and shape these Majorana modes. Recent studies have shown amazing advancement in this field and the fine-grained control required to create intricate quantum circuits. It’s like choreographing a difficult dance, every movement precisely regulated and directed toward a desired result. These Majorana modes, their non-Abelian statistics, provide a universe of possibilities for advanced quantum calculations right by themselves. Their special behavior could enable the building of quantum bits (qubits), which are naturally error-protected, therefore overcoming a main obstacle in quantum computing. One of main areas of attention is the development of quantum error correction methods. We could be able to design systems that are self-correcting, noisy and error resistant by deftly leveraging the features of these edge states.
Moreover, the study of topological superconductivity has offered interesting fresh directions of research bridging the gap between particle physics and condensed matter physics. The Majorana modes itself reflect a link to ideas derived from theoretical physics; the same mathematical formulations show in rather diverse domains. This surprising meeting offers fascinating new perspectives on the basic rules controlling the cosmos. The field is very rich and fulfilling because of its multidisciplinary character, which allows one to find new fundamental physics and simultaneously advance innovative technology. My continuous study includes investigating fresh material combinations and creating methods to improve the stability and controllability of Majorana modes. The ultimate aim is to build a dependable, scalable platform for quantum computing so launching a new era of technological innovation. A voyage of inquiry, the quantum dance at the edge pushes the envelope of our knowledge of the universe and uses its power for the good of all people. There is great possibility for discoveries in quantum protection, hence the dance keeps on.
Quantum Dance at the Edge

Have you ever dreamed of a time when quantum computers rival our laptops in dependability? That is the promise of “topological superconductivity,” an area that fascinates me absolutely. This is an amazing fusion of the very real realm of materials science with abstract particle physics, where the apparently impossible becomes concrete. My work focuses on the special behavior of electrons in some superconducting materials, a behavior that generates Majorana modes, exotic quantum states with the potential to transform quantum computing. These are not your typical particles; they show a unique quantum dance at the margins of these materials, a dance controlled by topology, a field of mathematics that explains forms and their characteristics. Stable and dependable quantum computers depend on this dance, which violates conventional wisdom. I find it really fascinating how basic physics relates to useful applications. We are designing and synthesizing fresh materials, stretching the envelope of what is feasible, not only investigating abstract ideas. To further the subject of quantum computing, my effort consists in precisely regulating these fragile Majorana modes and deciphering their behavior. This will signify what for the future? It implies a time when computation’s accuracy and power will leap ahead. Consider the opportunities for materials science, medicine, and many other disciplines.
The Intricate Dance: Unveiling Majorana Modes
With an eye on using Majorana modes, my studies go deeply into the nuances of “topological superconductivity.” We investigate fresh material combinations and designs to maximize their features. Consider it as controlling the movements of these strange particles to improve their performance, thereby choreographing the quantum dance. My group has produced a hybrid material combining a topological insulator, a substance with special surface qualities. Majorana mode stability improved remarkably as a result. Constructing this material required exact layer control via a method known as molecular beam epitaxy. To find the unique fingerprints of Majorana modes, we then carried tests at very low temperatures—think of near absolute zero! We quantized their conductance and observed their magnetic field response. Crucially, overcoming obstacles including keeping ultra-low temperatures and reducing noise required constant attention. Our new material’s Majorana modes were proven to exist and to be rather stable by the data we acquired. This represents a major step toward creating useful quantum tools. It wasn’t easy, though; we had to use sophisticated statistical techniques to isolate the faint signals from the background noise—like searching a very, very chilly haystack! Still, what comes next? To improve quantum computing features, we are investigating quantum entanglement and non-Abelian anyons. What are the ultimate ramifications of this investigation? Within our reach are fault-tolerant quantum computation and quantum error correction.
The Future of Quantum Computing: Challenges and Dreams
Study of topological superconductivity deftly combines particle physics with condensed matter physics. The Majorana modes themselves function as a link between ideas in theoretical physics. The multidisciplinary character of the field makes it immensely rich and fulfilling. We are developing transforming technologies and learning fresh fundamental physics at the same time. My work keeps looking at novel material combinations and developing sophisticated methods to increase the stability and control of Majorana modes. Our highest aim is To provide a dependable and scalable platform fit for quantum computing. There is great possibility for discoveries in quantum protection, a means of shielding quantum information from outside disruptions. Changing Majorana modes opens some amazing opportunities. In quantum information science, they hold the key to address several difficult issues. Still, there are major difficulties. Many modern systems, for instance, demand quite low temperatures. How might one fix this? Realizing topological quantum computing will depend on raising the operating temperature. Another major obstacle is exactly managing and manipulating these Majorana modes. Development of effective quantum error correction methods is absolutely vital. An ongoing trip of discovery, the quantum dance at the edge pushes the envelope of our knowledge and uses it for the good of mankind. Ready to see the revolution?
Quantum Dance at the Edge
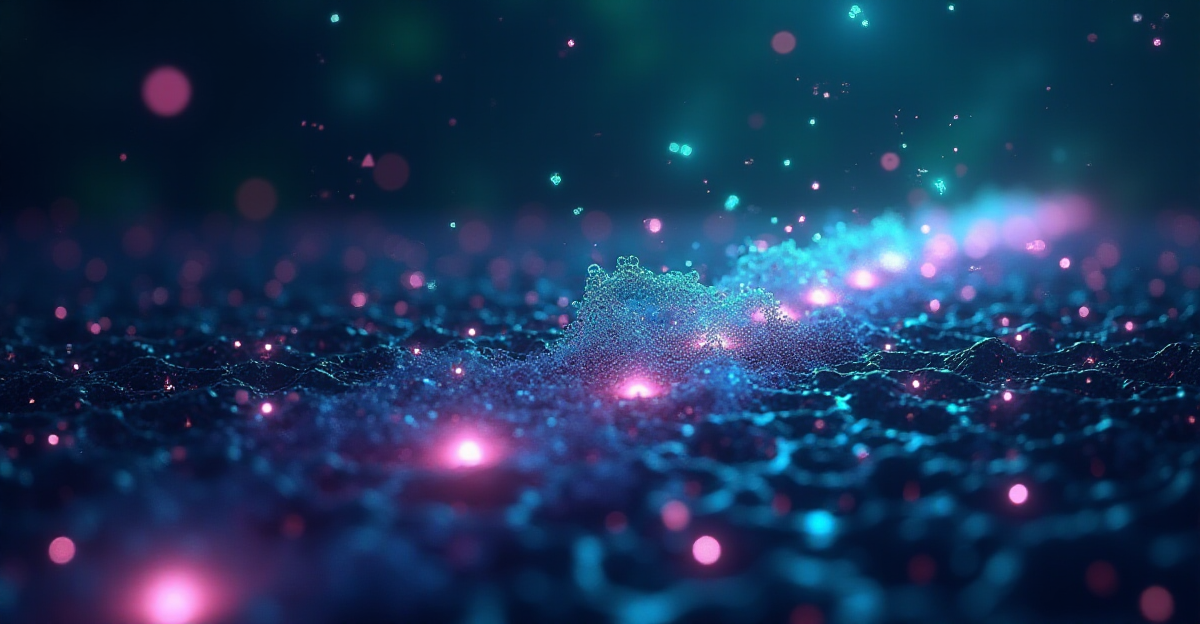
Imagine a world in which computers tackle formerly unachievable tasks at unthinkable rates. Quantum computing promises this, and my work is on topological superconductivity, a really interesting field. For years, the combination of abstract mathematics and the pragmatic reality of materials science captivated me. It revolves on the odd electron behavior in particular superconducting materials that produces Majorana modes—particles with their own antiparticles. Found near the margins of these materials, these extremely strong particles withstand the noise that afflicters conventional quantum systems. Building stable and dependable quantum computers able of very accurate computations depends on this robustness. From speeding drug discovery through exact simulations to generating exponentially quicker methods for challenging situations, the possible advantages are significant. My work always pushes the envelope of what we think feasible while exploring both the basic ideas and their applications. Applying topological superconductivity’s apparently theoretical elements to the design and synthesis of novel materials helps one to see them physically. This might transform several disciplines and has great consequences for quantum information science. The challenge of tying theory and practical application together appeals greatly to me. From theoretical ideas to practical implementations, the path of discovery and ongoing education is rich. Furthermore improving the experience is the cooperative character of scientific research.
Unlocking the Secrets of Majorana Zero Modes
Using the special features of Majorana zero modes, topological superconductivity presents a possible route towards fault-tolerant quantum computers. These particles, found at the margins of some materials, show non-Abelian statistics—that is, their order counts throughout interactions—a crucial characteristic for uses in quantum computers. To increase the stability and control of Majorana zero modes, my research group looks at new material combinations and architectures. We are actively developing their quantum shielding to reduce their sensitivity to outside disturbances possibly causing computational mistakes. For Majorana zero mode stability, for instance, we recently created a hybrid material using a topological insulator, greatly enhancing This included rigorous layer-by–layer control with molecular beam epitaxy and then ultra-low temperature investigations. By means of their quantized conductance and sensitivity to magnetic fields, the tests exposed the unique fingerprints of Majorana zero modes. Extensive statistical techniques were essential in separating these faint signals from background noise. We also investigate quantum entanglement actively in order to improve quantum computing capacity. Think about the possibility of accelerated drug development by use of exact simulations. Advances in topological quantum computing enable this and might significantly shorten development times and expenses. There are wide ramifications for fault-tolerant quantum computation and quantum error correction. Our present emphasis is on finding creative strategies to raise coherence times and enhance controllability as well as on deepening knowledge of Majorana zero mode behavior. There is great possibility to raise the speed and accuracy of simulations relevant for different scientific and technical issues.
Confronting the Challenges in Topological Quantum Computing
With Majorana zero modes acting as a link between theoretical predictions and practical findings, topological superconductivity wonderfully links particle physics and condensed matter physics. This multidisciplinary approach makes it really rich and fulfilling. Investigating new materials and innovative approaches to improve the stability and management of Majorana zero modes for a scalable and dependable quantum computing platform is part of my continuous research. One major obstacle is the requirement for very low temperatures in many of the present systems Making topological quantum computation a useful technology depends on raising the operational temperature. Still formidable challenges are precisely regulating and manipulating these Majorana zero modes and creating strong quantum error correcting techniques. Along with many other researchers worldwide, my team is committed to overcome these challenges and releasing the possibilities of topological quantum computing. This calls for investigating novel materials, honing manufacturing techniques, and creating sophisticated control systems. Success would transform disciplines including artificial intelligence and cryptography as well as medicine and materials science. The continuous investigation of topological superconductivity and the amazing features of Majorana zero modes will define the direction of quantum computation. Imagine creating a new medication; typically, this calls for years of research and improvement. Highly accurate simulations made possible by topological quantum computing will drastically save the time and resources required, therefore accelerating development and providing wider availability of life-saving medicines.
Extra’s:
For a deeper dive into the fascinating world of quantum phenomena and their applications in future technologies, you might find our post on “Quantum Mirages: Engineering Atomic Illusions for Future Computers” particularly insightful. This article explores how manipulating quantum states at the atomic level can lead to breakthroughs in computation, a concept closely related to the precise control of Majorana zero modes discussed in this piece. Furthermore, the intricate manipulation of energy levels and wave functions, central to understanding topological superconductors, shares parallels with the precision engineering required in “Phononic Frequency Combs: Sound Waves Meet Quantum Precision,” another exploration of quantum precision in a different physical domain. Both articles offer complementary perspectives on the challenges and opportunities presented by the quest for quantum technologies.
To expand your understanding of the theoretical foundations underpinning topological superconductors and Majorana zero modes, several excellent external resources are available. For instance, exploring reputable physics journals and university research papers can provide a wealth of information on the mathematical models and experimental techniques used in this field. You can also find numerous online courses and lectures covering the basics of condensed matter physics and topological insulators, which are essential for a comprehensive grasp of this subject. These resources offer detailed explanations of the underlying physics and can enrich your understanding of the complex concepts discussed in this blog post. Finally, reputable scientific review articles provide excellent summaries of the current state-of-the-art research in the field.
1 thought on “Edge States in Topological Superconductors: Dancing with Majorana Zero Modes”